Analysis of Film Extrusion Problems with Flow Simulation
- Published: September 30, 2004, By John Perdikoulias & Jiri Vlcek, Compuplast International Inc.
Email the author(s) at: jp@compuplast.ca.
Following is an expanded summary of a complete paper that is available on the TAPPI web site at tappi.org. On the page, click "the PLACE" in the section designated "Journals."
Application: Computer simulation can explain certain flow behavior and phenomena that can occur during film extrusion. This understanding can correct a problem and improve product quality.
Computer simulation has been applied to the film extrusion process and its equipment since the 1970s. Most film extrusion equipment manufacturers currently use some type of computer simulation to help ensure that the equipment will achieve the required performance. Initially, the main requirement for the simulation was to ensure a uniform distribution of the material for uniform product thickness. As understanding of the film extrusion process has improved, current simulations can now address more complex issues in film extrusion. This paper demonstrates the current capabilities of computer simulation by showing its applications for solving some film extrusion problems. The paper emphasizes the practical application of simulation.
Extruder
An extruder is a very challenging device to simulate because it incorporates several unit operations in one piece of equipment. The extruder conveys pellets, compresses them, melts them, mixes the melted material, exchanges heat with the barrel, and generates the required pressure to push the material through the system. Attempts at simulating the extrusion process have continued since the late 1950s. By the middle 1970s, adequate mathematical models were available. With the more commonly available computers in the late 1970s and early 1980s, simulation became more popular.
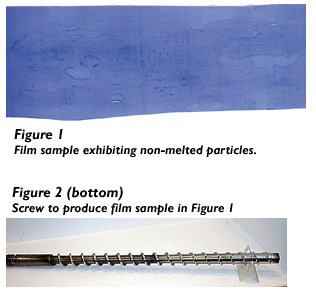
Initially, the extruder simulation focused primarily on predicting the output. With improvement in simulation, applications developed to understand process problems such as melt temperature, degradation or “gels”, surging, “un-melts,” and purge times.
Figure 1 is an image of a film sample with what were initially described as gels but later shown to be un-melted (or non-melted) particles. These particles are essentially polymer that has not properly homogenized. Figure 2 is a photograph of the screw used in this process. The discoloration on the surface indicates a problem in the first half of the screw.
In the feed section of a screw, the solid bed takes up the entire channel width and then generally becomes narrower as the material melts and forms the melt pool behind the solid bed. This behavior can be simulated and used as a criterion for evaluating screw performance. Simulating the screw design in Fig. 2 gave a plot of the solid bed ratio along the screw length.
The solid bed ratio represents the fraction of the channel that contains solid material along the screw length. It is an indication of the melting rate or melting capability of the design. Ideally, the solid bed ratio would start at 1.0 (100% solid material) in the feed section and gradually decrease to zero as the solid bed melts and the melt pool starts to form behind it. Sometimes, the solid bed width does not decrease continually and is predicted to increase. This “expansion” of the solid bed squeezes out the melt channel and results in an imbalance that can break the solid bed or result in a surge condition. The breakup of the solid or the surge generally means that particles of resin become encapsulated by the melted material and do not have exposure to the necessary shear stress to melt and mix properly. They then appear as “gels” — or more accurately “un-melts” — in the final product. The solid bed curve for this example increases at about 40%-50% along the screw length. This correlates with the end of the discoloration on the screw in Fig. 2. The compression of the screw channel happens too rapidly resulting in a restriction that “chokes” the material. Using this information, we would avoid using any screw design that gave a similar simulation result for solid bed profile.
Flat Dies
Simulation of a flat die is also very common, but most past simulations focused on obtaining a uniform distribution. This is a relatively easy criterion to achieve on its own, but it does become more difficult if the die must also satisfy other criteria. For example, a die should also have good “self-cleaning” or purging characteristics so that the dwell time of the material is minimized with concomitant decrease of polymer degradation. This is especially important if the degraded material becomes corrosive and can damage the surface.
A good criterion for avoiding this type of problem is the shear stress at the surface of the flow channels. Flat dies are generally prone to material degradation or long residence time in the manifold especially toward the edges of a die.
Spiral Mandrel Dies
A spiral mandrel distribution system has common use to achieve a uniform annular flow in a blown (tubular) film die. The flow in this type of distribution system is somewhat more complex than that in a flat die. This means spiral dies require more complex simulations.
As with flat die simulations, the primary focus in the past was to obtain a uniform flow of material around the circumference of the die. The shear stress criterion for adequate “self-cleaning” behavior is also important in these types of dies. In addition, the way in which the material distributes itself can result in a particular flow phenomenon that three-dimensional finite element method (FEM) simulation discovered. The phenomenon appears as a visual defect in a film bubble in the form of a faint, extrusion (machine) direction line. These lines are equal to the number of spirals and generally look like a weld line in the film. A three-dimensional FEM simulation performed on a die geometry that exhibited this phenomenon gave an understanding of the origin.
Simulation indicates how material from a particular spiral channel distributes itself around the circumference of the die. Some material from one spiral channel actually flows up and back down the previous channel opposite to the expected flow direction. This “back-flow” behavior essentially results in two opposing flow fronts merging to form a weld line with a flow stagnation point. The source of this phenomenon would have been very difficult to determine without the aid of the three dimensional FEM analysis.
Conclusion
This paper demonstrates how current simulation technology can help explain and ultimately solve certain problems that appear during film extrusion. In all the cases presented, the simulation helped to quantify a problem and establish criteria for later use to improve the process conditions or equipment design and correct the problem.